Making biomass available to meet long-term market demand

Biomass potential

Biomass potentials are difficult to estimate. Most estimates of future biomass potential for bioenergy rely on the scenario approach; this is based on different scenario parameters that have an impact on the target value. Thus, the overall range between biomass potential estimates is extremely wide: in the most extreme scenario, the theoretical biomass potential for bioenergy reaches over 1,500 EJ per year. This is equivalent to almost three times the present global primary energy supply. The sustainable implementation potential is estimated at between 60 and 120 EJ per year (corresponding to 9–18% of the total world energy supply).
Available biomass feedstocks
Currently available biomass feedstocks include dedicated energy crops from sustainable agriculture (preferably produced on abandoned, degraded, or marginal lands), residues from forestry and agriculture, various organic residue streams from wood and agro-food industries, as well as organic fractions of municipal waste. As a biological resource, algae could potentially provide a sustainable contribution for biomaterials and bioenergy production in the future.

Residue feedstocks from various agriculture sectors come in the form of i) primary crop residues such as rice husk, wheat straw, or manure; ii) sugar/starch and biofuel crops such as sugarcane, corn, rapeseed, soy, oil palm, or iii) lignocellulosic crops, such as short rotation coppice or energy grasses. Besides agriculture, forestry is the major biomass supply sector. Woody biomass from forests includes residues that otherwise would remain in the forest after harvests, (parts of) trees (thinnings, badly shaped stems, diseased trees) that are of no interest to conventional wood processing industries or as actual wood processing residues in these industries. Secondary residues are those from food, feed, and material production processes. Tertiary residues (e.g., post-consumer material such as demolition wood and scrap pallets) are another important feedstock source in terms of cascading use.
When using these biomass resources, we must also consider ecological criteria and the balance with carbon sinks.
Biomass demand for energy production
Of all renewable energy resources, biomass contributes the highest share to the global energy supply. Bioenergy, including the traditional use of biomass (burning of woody biomass or charcoal and agricultural residues in simple and inefficient devices in developing and emerging economies), contributed an estimated 12%, or 45.2 EJ, to total final energy consumption in 2018. This is equivalent to only 50% of the estimated mean sustainable biomass potential (90 EJ). Modern bioenergy, which excludes the traditional use of biomass in simple and inefficient devices such as three-stone fires without chimneys, provided an estimated 19.3 EJ—or 5.1% of total global final energy demand—in 2018.
In the stated policies scenario of the IEA global bioenergy demand assessment, the demand for solid biomass for traditional use will decrease to 23 EJ until 2040. In contrast, the demand for solid biomass for modern use will increase to 39 EJ. The 2040 demand for biofuels will increase to 9.4 EJ and for biogas, including biomethane, to 6.3 EJ. More ambitious pathways, however, that strive for net zero CO2 emissions by 2050, include the phasing out of traditional bioenergy completely by 2030, thereby partly amplifying the need for modern bioenergy.
Dynamic factors defining biomass availability
In practice, the availability of biomass for energy will be influenced by population growth, diet, water availability, farming and forestry practices, agricultural density, nature conservation, and (changing) climatic circumstances. No matter what type of sustainable biomass is considered or where it comes from, strategies for biomass availability would generally cover the following considerations:
- An agile and flexible large-scale biomass business case will be able to adapt to multiple sources of feedstocks, and continually move up the technological learning curve through learning-bydoing;
- Understanding of the biomass supply including the amount, locations, and quality;
- Realisation of the best value by connecting the biomass to the right market and use; and
- Scaling and integration with existing supply chains.
However, the availability of biomass resources and residues is mainly determined by future developments in agriculture and forestry. Aspects include availability of land for agricultural crops, agricultural practice, forestry practice, and demand for bio-based products with related biomass residues. Policy incentives and restrictions, such as sustainability requirements, will have a major impact.
Agricultural biomass is seasonal
These challenges can be partially addressed by innovative supply chain design. There could, for example, be a focus on establishing transport and storage systems for various types of agricultural biomass resources, or supply chains could also be managed through adapted technology solutions, for example, processing biomass to make it compatible with existing infrastructure.
The future supply of agricultural biomass is further limited by the biomass yield levels that can be obtained from the available land and what crop is chosen, the sustainability constraints imposed on bioenergy crop production and population growth, and the resulting food and feed demand.
To ensure future sustainable supply of agricultural residues as well as energy crops, the efficiency of feedstock provision processes and logistics, including the efficient collection of residues, should be optimised. There should also be a focus on modernisation and technology development in agriculture, including productivity increases and technology exchange, as well as the improvement of production of short rotation forestry and bioenergy crops (such as energy grasses and reeds) on marginal lands.
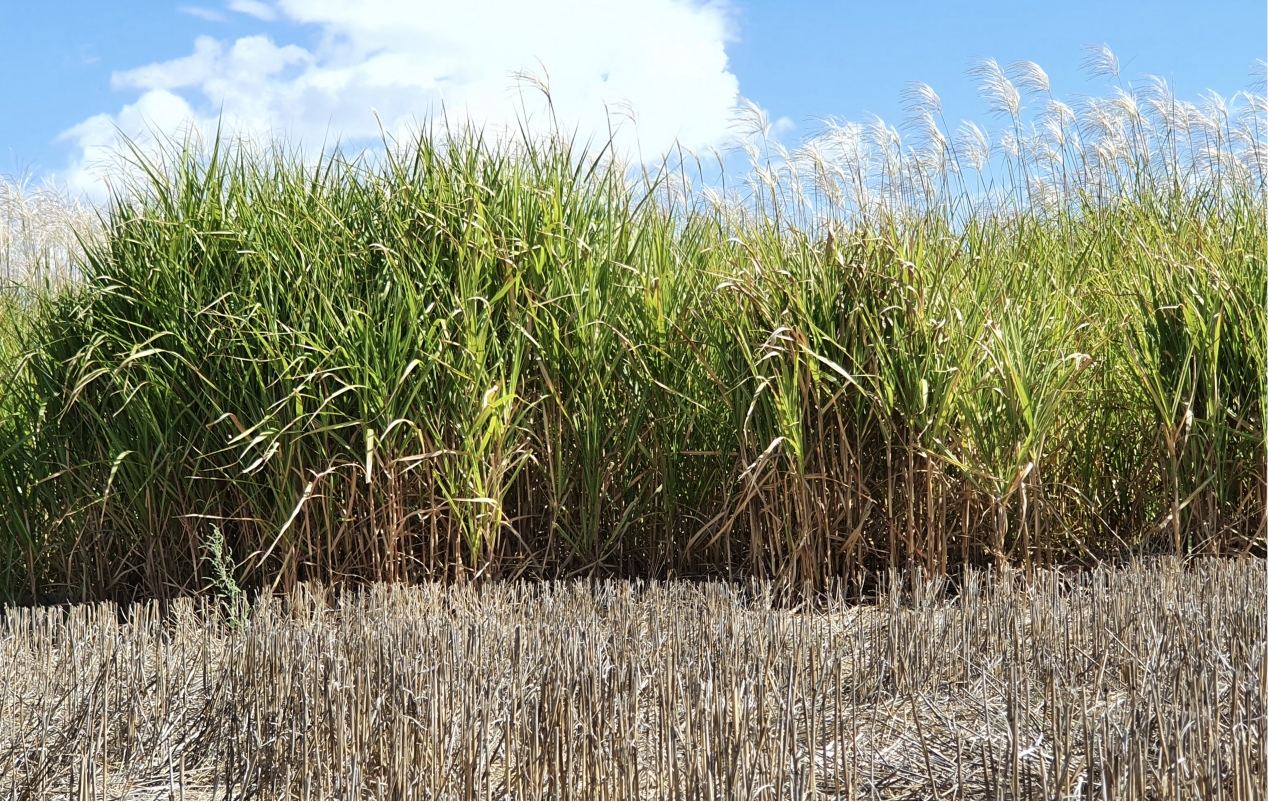
Forest biomass supply is inherently complex
Different types of biomass need to be accorded an appropriate value market. If there is a well planned supply chain management, specific local market needs can be met, and risks of failure can be decreased if local conditions are considered. Forest biomass supply chains need to continuously adapt to changing conditions such as the opening and closing of saw mills or pulp and paper mills, as well as to catastrophic circumstances.
Waste materials
For used cooking oil (UCO) or organic municipal solid waste (MSW), the core logistical challenge is the collection and management of these wastes. Consumer awareness and behaviour play an important role in facilitating the set-up of such biomass value chains. Finally, safety and hygiene, and regulatory aspects must also be considered. The growing global demand for FAME biodiesel and Hydrogenation-Derived Renewable Diesel (HDRD or HVO), coupled with policy incentives to use waste feedstocks, have significantly improved UCO collection in some regions such as North America and the EU.
Algae biomass
Biomass mobilisation - Possibilities for enhancing biomass availability
More development is needed to mobilise biomass potentials and to address risks and uncertainties associated with feedstock costs, its quality and quantity, as well as supply chain efficiency. IEA Bioenergy scientists and industry experts agree that this requires focus on legislative frameworks, technological innovation, and market creation.
The legislative framework dimension explores the current policy landscape and connects the different policy strategies, blueprints, roadmaps, action plans, and funding schemes. Multi- level governance concepts provide a framework for acknowledging the interactions between the different spatial or organisational resolutions. Participatory processes in governance will be decisive in terms of social acceptance. The remarkably high societal participation potential for circular bioeconomy supply chains must be acknowledged to empower energy democracy and harness multiple societal benefits.
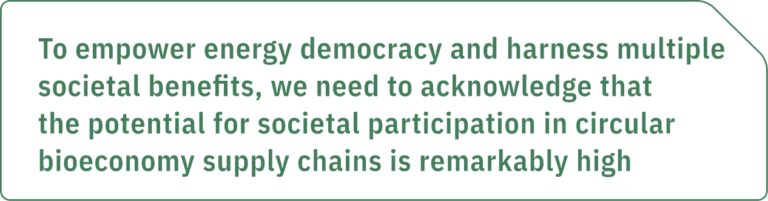
The market creation dimension focuses on market catalysts for wastes, residues, post-consumer products, and secondary raw materials. Moreover, market platforms such as bio-hubs should be addressed.
Bio-hubs are making a variety of biomass types available at a single location. The key advantages of bio-hubs include the streamlining of processing, storage, and transportation, reduction in administrative costs, providing an opportunity for suppliers of biomass products to continue producing in the off-season (e.g., in the summer, when residents or building occupants do not require heat), and providing a place for companies to connect and trade with one another. Biohubs also provide a sales point for local biomass residues.
Biomass supply via bio-hubs as an alternative to the conventional supply route provides opportunities to manage quality, support new production, and develop critical logistical mass through biomass uniting and pre-processing hubs. As bio-hubs have the potential to improve the security of biomass supply, they would be able to mobilise less profitable biomass and take this market to the next level.
To minimise risks related to the operation of bio-hubs, it would be advisable to either start small or to build on the existing business cases or facilities. This approach would still allow businesses to grow to a larger-scale operation with multiple suppliers and a diversified portfolio of products, depending on the loca tion.
Mobilisation level “technological innovation”, refers to quite specific developments such as mobile and portable pre-treatment processes and precision farming/forestry based on planning and harvesting supported by geographic information (GIS) systems; this includes models, services, and start-ups. Science and evidence-based integration of bio-based supply chains with other renewable energy sources—that is, in a broader sustainable economy—will provide resilience for an economic metabolism under changing framework conditions.
Pre-treatment of biomass residues can improve and enable supply chains for thermochemical conversion. Pre-treatment results in fuels with better defined specifications and increased energy densities. Through pre-treatment steps, such as washing, drying, sieving, leaching, or thermal pre-treatment, the characteristics of lower-grade biomass can be significantly improved and, in some cases, will also more closely mimic the characteristics of the fossil fuel for which it is being substituted. Increased volumetric energy density decreases feedstock transportation costs and logistical challenges. The IEA Bioenergy report “Biomass pre-treatment for bioenergy” has analysed five individual case studies, including biomass torrefaction, pre-treatment of woody residues, treatment of municipal solid waste (MSW) to produce solid recovered fuel (SRF), steam explosion for co-firing, and leaching of herbaceous biomass.
- Offermann R, Seidenberger T et al. (2011) Assessment of global bioenergy potentials. Mitigation and Adaptation Strategies for Global Change 16, 103–115 LINK
- Searle S, Malins C (2015) A reassessment of global bioenergy potential in 2050. GCB Bioenergy 7, 328–336, LINK
- REN 21, Renewables 2020 global status report LINK accessed 18/02/2022
- IEA, Breakdown of global bioenergy demand in the Stated Policies Scenario, 2010-2040 LINK
- IEA (2021) Net Zero by 2050 – A Roadmap for the Global Energy Sector LINK accessed 18/02/2022
- IEA Bioenergy Task 43 (2018) Innovative approaches for mobilization of forest biomass for bioenergy LINK accessed 18/02/2022
- EU Science Hub, The European Commission’s science and knowledge service (2020) Algae biomass production for the bioeconomy LINK
- Tattersall Smith C, Lattimore B, et al. (2017) Opportunities to encourage mobilization of sustainable bioenergy supply chains WIREs Energy and Environment, 6:e237. LINK
- IEA Bioenergy Task 43 (2017 ) Mobilisation of agricultural residues for bioenergy and higher value bio-products: resources, barriers and sustainability LINK accessed 20/06/2022
- IEA Bioenergy Task 43 (2019) Bio-hubs as keys to successful biomass supply integration for bioenergy within the bioeconomy LINK accessed 18/02/2022
- IEA Bioenergy (2019) Fuel treatment of biomass residues in the supply chain for thermal conversion LINK accessed 18/02/2022
Residues as resources—bioenergy instead of decomposition
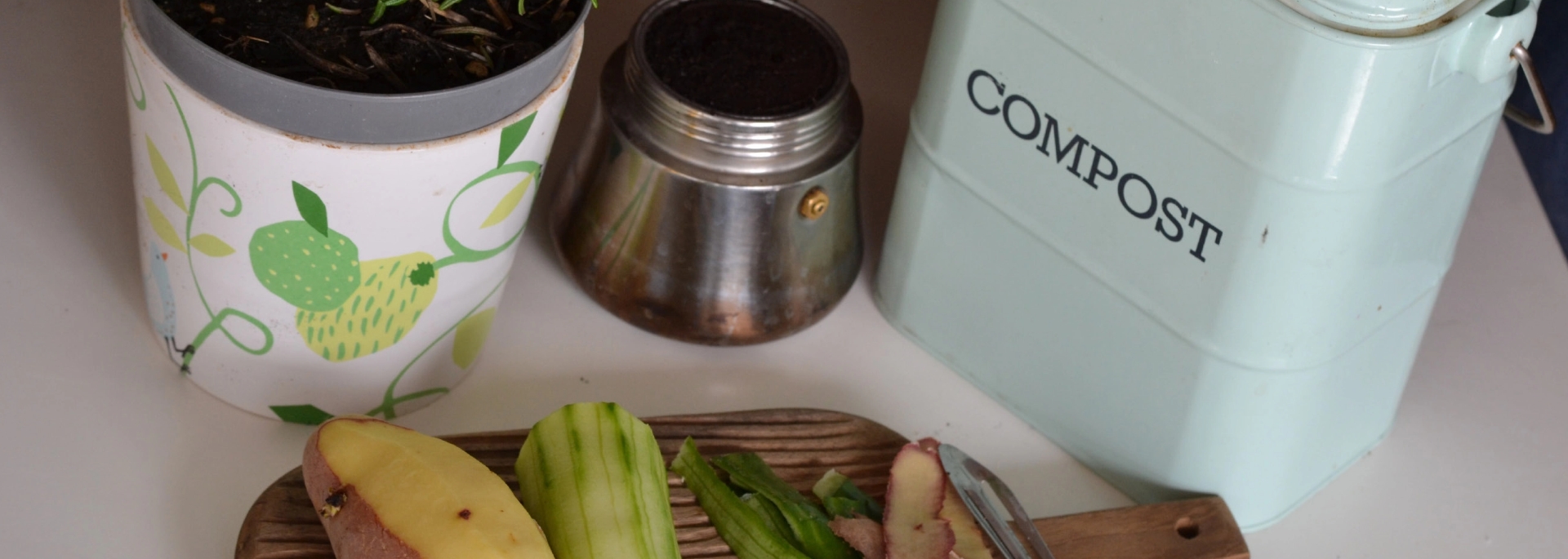
There are different sources of biomass residues: those remaining from the harvest of trees, agriculture residues left in the field after harvesting or pruning, those discarded during the processing phase (for instance, olive pits and nutshells), residues from wood and food industries, farming and livestock manure, the organic part of municipal solid waste, and sludge from sewage treatment plants. The common practices of natural biomass waste decomposition or the open field burning of agricultural residues can cause unhealthy air pollution as well as high risks of fire escaping into forests and endangering health and life.
In India and other Asian countries, farmers often burn rice straw in the fields, with the aim of removing residues and preparing the field for the next crop. This practice leads to an uncontrolled combustion process which has a high impact on air quality, with emissions of particulate matter (PM), polycyclic aromatic hydrocarbons (PAHs), nitric oxides (NOx) and other pollutants.
South Africa, like many other countries in the African continent, discards over 90% of municipal solid waste and industrial biomass onto the land, without any effective recycling of materials or resources.
Approaches to utilising biomass residues and waste fractions
Biomass residue streams and waste fractions are potential feedstocks for a variety of products, including biofuels for transport, chemicals, and construction materials.
In well developed economies, a cascading use of biomass is at the core of a circular economy, which means that residues and recycled materials are used efficiently to extend total biomass availability within a given system. The cascade chain would allocate high-quality woody biomass first to long-lived products, such as building materials, then to refurbishment, and eventually to re-use and recycling, finally putting it to energetic use before it ends up in landfill disposal.

Improving the cascading and resource-efficient use of biomass requires interventions throughout the whole value chain. There is no one-size-fits-all solution to improve resource efficiency and implement the cascading use of biomass. Only a mix of coordinated approaches—tailored to the local specifics—will lead to full implementation. It should be noted that wherever access to biomass for priority services such as heating and cooking is low, the use of high-quality woody biomass for heating and cooking may still take priority over its use for products of higher economic value.
- Solid recovered fuels are produced from individual or mixed streams of wastes from different sectors (municipal, commercial, industrial, construction, demolition). These streams include plastic, paper and cardboard, textile, and wood and biogenic fractions. Mechanical or mechanical-biological treatments are used to produce solid recovered fuels. These fuels are in high demand by energy-intensive industrial sectors (like the cement sector) as substitutes for fossil fuels. Solid recovered fuels may have great potential in countries that have to manage
high annual amounts of wastes (such as China and India) while also satisfying their internal energy demand.
- Manure and sewage sludge can be reused as fertilisers or for the recovery of highly valuable phosphorus via advanced processes. Care needs to be taken, however, not to contaminate farmland with heavy metals and pharmaceutical residues from the sludge.
At the stage of energy valorisation, the main established processes for converting biomass residues into bioenergy are combustion and biological treatment.
- Combustion of biomass residues from forestry and agriculture produces heat and electricity. For example, in district heating systems, locally produced woody residues (wood chips and sawdust) are combusted to supply heating and domestic hot water to communities.
- Anaerobic digestion of manure, organic industry waste, and a separately collected organic fraction of municipal solid waste produce biogas which is used either directly for heat and/ or power generation, or upgraded to biomethane and further fed into the gas grid. The second product of the process is a digestate that can be used as a biofertiliser.
- Biomass residues such as straw and corn cobs can be fermented into ethanol for use as transport biofuel. While the production of ethanol from sugar and starch crops has reached maturity, technologies for producing ethanol from forestry and agricultural residues and waste materials are currently under development.
- Gasification converts solid materials (biomass, as well as other fractions of municipal solid waste such as paper, cardboard and textiles) into a combustible gas (syngas). This gas can be used for the synthesis of fuels (such as hydrogen, methane, and others), for chemical products, or to generate heat and/or electricity. When applied to biomass-based materials, gasification can produce energy with low carbon intensity, as successfully demonstrated at several plants in Europe. Researchers in the scientific community are working to further improve the process and to explore new pathways for the production and use of biofuels and chemicals.
- Pyrolysis is used to produce liquid oil that can be either directly combusted to produce heat or upgraded into transport biofuels. Additional products of pyrolysis are gases and also biochar.
- Biomass residues can also be liquified by hydrothermal liquefaction to produce a liquid “biocrude” that can be upgraded into transport biofuels or valuable chemical products.

Biomass residues are valuable feedstocks that can help contribute to climate change mitigation by displacing substantial amounts of fossil fuels and carbon-intensive materials, thus reducing GHG emissions. The IEA Net Zero by 2050 Roadmap estimated that biomass residues could provide an energy supply of around 65 EJ/year by 2050 (20 EJ from wood processing and forest harvesting and 45 EJ from agricultural and food processing residues, as well as industrial and municipal organic waste streams). Another study analysed quantities of biomass residues supplied for energy and their sensitivities in harmonised bioenergy-demand scenarios and found that residues could meet 7–50% of bioenergy demand towards 2050 (depending on different scenarios).
- Gadde B, Bonnet S et al. (2009) Air pollutant emissions from rice straw open field burning in India, Thailand and the Philippines, Environmental Pollution 157 1554–1558 LINK
- Kim Oanh NT, Tipayarom A et al. (2015) Characterization of gaseous and semivolatile organic compounds emitted from field burning of rice straw, Atmospheric Environment,119, 182-191, LINK
- Sillapapiromsuk S, Chantara S et al. (2013) Determination of PM10 and its ion composition emitted from biomass burning in the chamber for estimation of open burning emissions, Chemosphere, 93, 9, 1912-1919 LINK
- IEA Bioenergy Task 36 (2021) Transitioning towards a decarbonised circular economy: Focus on Waste to Energy LINK
- Vis M, Mantau U, Allen B (Eds.) (2016) Study on the optimised cascading use of wood. No 394/PP/ENT/RCH/14/7689. Final report. Brussels 2016. 337 pages LINK accessed 18/02/2022
- IEA Bioenergy Task 36 (2020) Trends in the use of solid recovered fuels. LINK accessed 18/02/2022
- IEA Bioenergy Task 36 (2019) Nutrient recovery from Waste. LINK accessed 18/02/2022
- IEA Bioenergy Task 36 LINK accessed 18/02/2022
- IEA Bioenergy Task 32 (2019) Best practice report on decentralized biomass fired CHP plants and status of biomass fired small- and micro scale CHP technologies.LINK accessed 18/02/2022
- Nussbaumer T, Thalmann S, prepared for IEA Bioenergy Task 32 (2014) Status Report on District Heating Systems in IEA Countries LINK accessed 18/02/2022
- IEA Bioenergy Task 37, Energy from Biogas LINK accessed 20/06/2022
- IEA Bioenergy Task 36 (2019). Biomass pre-treatment for bioenergy. Case study 3: MSW pre-treatment for gasification LINK accessed 20/06/2022
- IEA Bioenergy Task 33 Projects LINK accessed 18/02/2022
- IEA Bioenergy Task 34, Pyrolysis Principles LINK accessed 18/02/2022
- IEA Bioenergy Task 34, Solvent liquefaction LINK accessed 18/02/2022
- IEA (2021) Net Zero by 2050 – A Roadmap for the Global Energy Sector LINK accessed 18/02/2022
FURTHER READING
- Gadde B, Bonnet S et al. (2009) Air pollutant emissions from rice straw open field burning in India, Thailand and the Philippines, Environmental Pollution 157 1554–1558 LINK
- Tripathi N, Hills CD et al. (2019) Biomass waste utilisation in low-carbon products: harnessing a major potential resource. npj Climate and Atmospheric Science 2, 35 LINK
- V. Hanssen S, Daioglou V et al. (2019) Biomass residues as twenty-first century bioenergy feedstock—a comparison of eight integrated assessment models, Climatic Change volume 163, 1569–1586 LINK
- UNEP (2009) Converting Waste Agricultural Biomass into a Resource – Compendium of Technologies LINK accessed 24/06/2022
- Biomass feedstock costs and prices FAOSTAT, LINK accessed 18/02/2022
- IEA Bioenergy, Annual Report 2020 LINK accessed 18/02/2022
- Fuhrmann M, Dißauer C, et al. (2021) Analysing price cointegration of sawmill by-products in the forest-based sector in Austria. Forest Policy and Economics 131, 102560, LINK
- Kristöfel C, Strasser C et al. (2014) Analysis of woody biomass commodity price volatility in Austria, Biomass and Bioenergy 65, 112-124 LINK
- Brosowski A, Thrän D et al. (2016) A review of biomass potential and current utilisation – St atus quo f or 93 biog enic wastes and residues in Germany. Biomass and Bioenergy 95, 257-272 LINK
Biomass feedstock costs and prices

Biomass costs
Reliable supply of affordable biomass feedstocks is crucial for the development of sustainable bio-based businesses. It is needed to reduce financial, technical, and operational risk to biomass supply and conversion investments. The additional risks associated with the long-term supply of affordable biomass feedstock having to meet appropriate sustainability criteria, are a significant complicating factor for financing bioenergy projects.
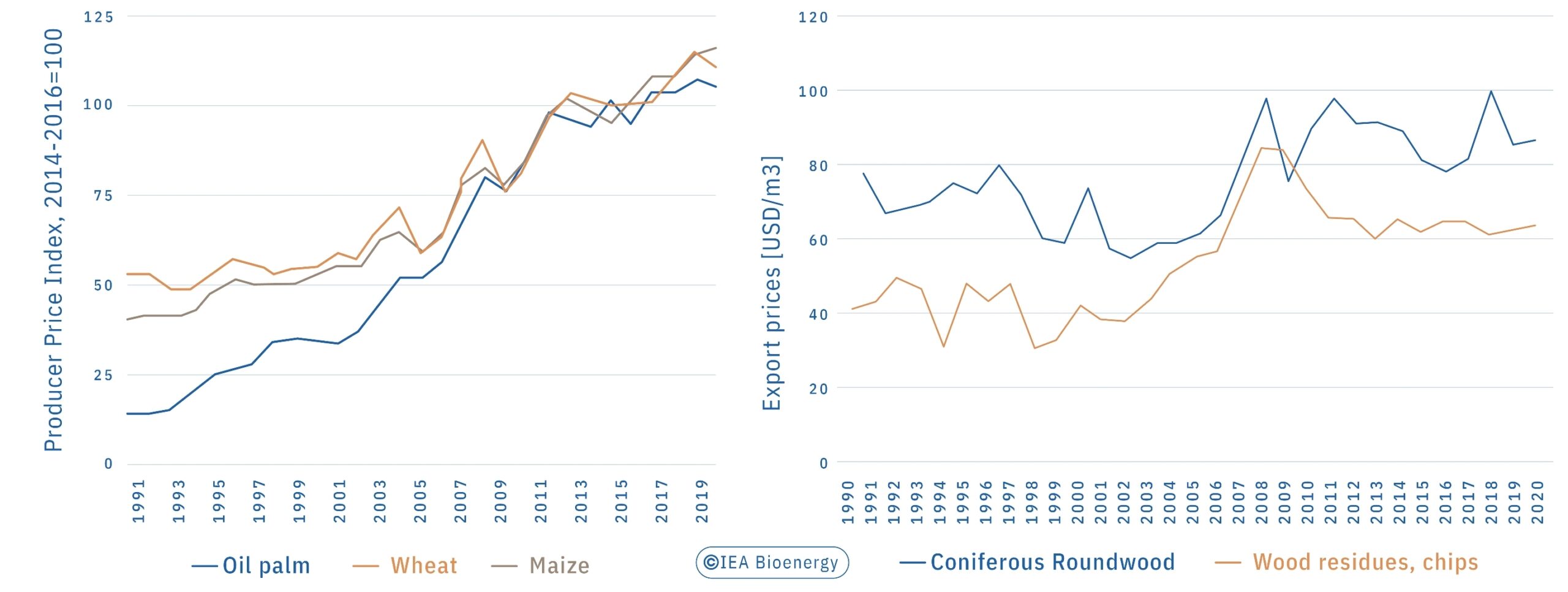
Right side: Nominal c oniferous roundwood and w ood residues, chip expor t prices in $/ m3 from 1990 t o 2020, Source: FAOSTAT
- Cultivation costs such as seed, fertiliser, irrigation water, labour and machinery time, etc. The costs depend on the biomass and the regional economic, topographical, and climatic conditions. If residues are used, cultivation costs can be disregarded.
- Costs for harvest and collection, storage, pre-processing, transportation and handling.
Harvesting, transportation, and handling costs for biomass in its different forms vary due to individual characteristics of different biomass feedstocks, quantities removed, distance to the using/storage facility, terrain, road condition, and other considerations. There is a tremendous variation in these factors within different regions. Biomass harvesting, transportation, and delivery systems must therefore be designed to meet constraints at the local level. Improvements in feedstock productivity and biomass harvesting, processing, and logistic systems could further reduce the biomass production cost. In addition, the economic efficient use of currently under- utilised biomass resources should be supported by the development of cost-efficient supply chains.
Biomass prices and markets
Whereas biomass production costs are expected to decrease due to biomass supply chain optimisation, biomass prices are expected to rise due to the following biomass market developments: i) the demand for biomass along well established value chains is increasing (e.g., demand for sawmill by-products); and ii) innovations and new technologies such as the production of biomethane and advanced biofuels are attracting new actors to enter the biomass market.
As a result, we see an increasing competition for biomass feedstock and, given that supply is limited, this is leading to rising prices. In addition, a higher demand incentivises suppliers to provide more raw materials. A simultaneous increase in raw material supply and demand can balance these effects, as prices are likely to decrease or stagnate, respectively.

Naturally, industries strive to use the cheapest raw material possible. The competition, particularly for by-products and residues, is thus likely to increase further. Price dependencies are therefore of interest when it comes to assessing the extent of influences on the bioenergy production cost.
Analysing and understanding biomass price volatility behaviour allows cost-effective supply chain management, including in the import and export trade. At the microeconomic level, biomass feedstock price volatility is relevant for the assessment of investment returns and capacities .
Biomass market development
Increasing fossil fuel prices have made a growing number of agricultural feedstocks competitive as feedstocks for the energy market. The extra demand has resulted in a global rise in agricultural commodity prices. The demand shift from traditional agricultural commodities to agricultural residues such as straw and forest-based feedstocks for bioenergy production should, however, result in falling real prices.
There are two types of wood residue that are of particular interest as feedstock: logging residues and industrial by-products that are mainly provided by the sawmill industry. The availability of logging residues is limited by the amounts that need to stay in the forest to manage the soil nutrient balance; moreover, they are difficult to collect as they are spread across the forest; quality criteria, like ash or energy content, restrict their use in some applications. In contrast, sawdust, wood chips, and bark ar e sawmill by-products with no additional procurement costs.
Biomass from forest tree species can also be produced by intensive cultivation of fast-growing species (usually willow and poplar) in short rotation coppice (SRC). SCR has a shorter investment payback period. Due to intensive production, however, the presence of only one or a few genotypes and artificial regeneration, such plantations are more vulnerable to natural disturbances. Hence, higher discount rates need to be considered in economic analyses.
With respect to using “biomass waste” as feedstock, a transition from waste to valuable resource can be expected, reducing the need to pay for disposal. The efficient use of currently underutilised biogenic by-products, residues, and wastes plays a key role in a system using bioenergy. However, the market conditions are still rather immature compared to fossil fuels. To access this particular biomass potential, efficient supply chains need to be established and regulations must be clarified, such as the management of sustainability criteria.
- Gadde B, Bonnet S et al. (2009) Air pollutant emissions from rice straw open field burning in India, Thailand and the Philippines, Environmental Pollution 157 1554–1558 LINK
- FAOSTAT LINK accessed 18/02/2022
- IEA Bioenergy, Annual Report 2020 LINK accessed 18/02/2022
- Fuhrmann M, Dißauer C, et al. (2021) Analysing price cointegration of sawmill by-products in the forest-based sector in Austria. Forest Policy and Economics 131, 102560, LINK
- Kristöfel C, Strasser C et al. (2014) Analysis of woody biomass commodity price volatility in Austria, Biomass and Bioenergy 65, 112-124 LINK
- Brosowski A, Thrän D et al. (2016) A review of biomass potential and current utilisation – St atus quo f or 93 biog enic wastes and residues in Germany. Biomass and Bioenergy 95, 257-272 LINK