Biogas production
Biogas is largely produced from different types of residues and by-products: biodegradable wastes (e.g., organic fraction from municipal solid waste), industrial by-products/wastes (e.g., food and beverage industry), agricultural by-products (manure, straw), and wastewater (sewage sludge, industrial wastewaters). In some specific countries, biogas is produced from purposely grown biomass feedstock, for example, maize or so-called cover crops, which allow an additional crop to be harvested on an agricultural cultivation area.

Manure from farm animals is one of the various substrates usable for biogas production (Photo credit:Pexels/Mail Maeder
Biogas is largely produced from different types of residues and by-products: biodegradable wastes (e.g., organic fraction from municipal solid waste), industrial by-products/wastes (e.g., food and beverage industry), agricultural by-products (manure, straw), and wastewater (sewage sludge, industrial wastewaters). In some specific countries, biogas is produced from purposely grown biomass feedstock, for example, maize or so-called cover crops, which allow an additional crop to be harvested on an agricultural cultivation area.
Biogas can be used locally for heating or for combined heat and power production. Alternatively, energy producers can upgrade biogas to biomethane. This upgrading includes removal of impurities and carbon dioxide from the gas. The greatest effort lies in removing the carbon dioxide due to its high concentration (typically 30–40%). The most commonly applied technologies for gas cleaning are membrane separation, water or chemical scrubbers, and pressure swing absorption (PSA). Once the CO2 is removed, the final product is called biomethane which has similar properties to natural gas, allowing it to be directly substituted for natural gas and using the existing infrastructure and utilisation technologies. Biomethane can be injected into gas grids or directly passed on to customers (e.g., local fuel stations); it also increases the share of green gas distributed to customers. Depending on local regulations, biomethane might need the addition of propane to adapt its calorific value and odorification when it is injected into the grid.
After the anaerobic digestion process, undegradable biomass, nutrients, and water remain as the non-gaseous by-products. This fermentation residue is called digestate. In most cases it is liquid; however, after, for example, dry digestion processes or centrifugation, solid digestates are produced. These digestates (either liquid or solid) are ideally utilised as fertilisers and applied on land in a circular economy approach. To enable this, the digestate has to meet certain quality requirements (regarding e.g., heavy metal content), which are mainly influenced by the
feedstock quality.
Finally, biogas plants can provide versatility and flexibility to the energy system: versatility because they produce heat, electricity, and biomethane; and system because they can be stored: raw biogas (on-site), upgraded biomethane (gas grid), heat (heat storage). In addition, the CO2 separated during biogas upgrading can be seen as another product and utilised as CO2 fertiliser in horticulture or in future in methanation and power-to-gas concepts.

Technology readiness level and status of implementation
Anaerobic digestion for the production of biogas is a very well established, mature technology (TRL 9) and is applied worldwide. It has a long tradition, with simple approaches having been applied for wastewater and sewage treatment for thousands of years ago. More industrialised and sophisticated approaches were developed at the end of the 19th and beginning of the 20th century after the introduction of septic tanks and Imhoff tanks for wastewater treatment.

Today, biogas technology has various fields of application:
Wastewater treatment: Biogas applications are used for the biological stabilisation of sewage sludge and the treatment/pollution reduction of high strength industrial wastewaters. In both cases, energy recovery by biogas is a welcome side-effect.
Waste treatment: In municipalities the driver for biogas plants is the treatment of food waste and bio-waste (e.g., the organic fraction of municipal solid waste). Wherever such wastes are landfilled, an (uncontrolled) anaerobic degradation process also takes place and the landfill gas can be recovered (if the landfill has been designed to accommodate this); this is comparable to biogas, although it also contains other types of impurities. In different industries (food and beverage, biofuel) residues and by-products accumulate which can also be treated in a biogas plant for energy recovery.
Agricultural applications: Agricultural residues (manure, straw, harvesting residues) can be treated in biogas plants. Energy crops (e.g., maize) are also used as biogas feedstocks in countries such as Germany, although this has recently been on the decrease.
Environmental effects

- Biogas (or biomethane) directly replaces fossil gas in industry or in CHP units. Biomethane also replaces fossil fuels in the transport sector.
- Waste Management: Including biogas plants in the waste management system drives separate collection of organic wastes and reduces direct landfilling.
- Methane emission reduction: The treatment of specific feedstocks can reduce the emissions of methane which is a very potent GHG. Treating manure in a biogas plant can avoid methane emissions from manure storage. Recovering and using the gas produced in landfills and treating organic wastes in a biogas plant rather than sending these wastes to landfill also have a very positive environmental effect.
- Air pollution: The combustion of biomethane in internal combustion engines releases fewer local air pollutant emissions than fossil fuels.
- Nutrient management is an essential part of biogas concepts. Nitrogen and phosphorous emissions to groundwater or surface water can be reduced by good practice in digestate handling, thereby reducing eutrophication.
- Digestate as biofertiliser replaces fossil-based fertilisers. Stabilised organic matter is also returned to the soil, and this can act as a carbon sink.
To guarantee that biogas plants will have positive environmental effects, it is essential to ensure minimum fugitive emissions from the process. Emissions during the combustion of biogas (e.g., NOx) should be kept to a minimum.
Costs
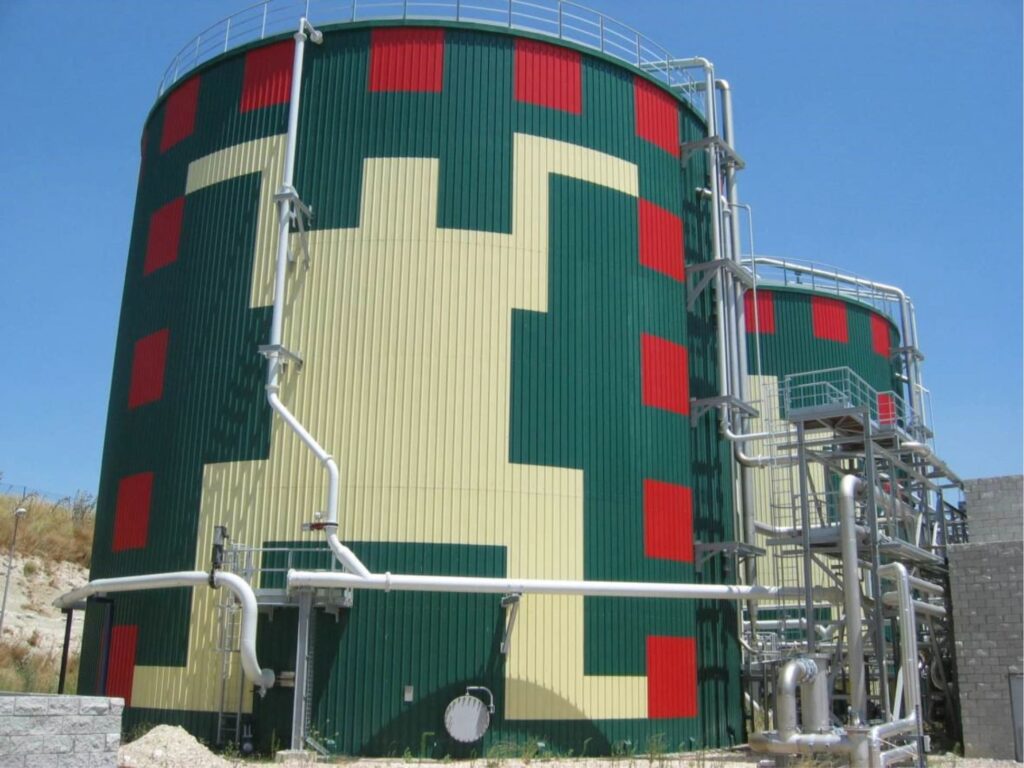
The costs of biogas production can be categorised as follows: investment costs (depreciation costs), feedstock costs, staff costs, maintenance costs, and other running costs.
Feedstock costs: If wastes are used as a feedstock, a charge—a gate fee, for example—is usually levied for treating the wastes, thus producing revenue. While industrial residues and by-products can be very cheap to use as feedstocks, other products, like fermented energy crops, can be very costly. There is thus a wide range of possible feedstock costs, and these are highly dependent on the specific biogas project and the national boundary conditions. Investment costs: Investment costs (depreciation costs) including peripheral equipment and installations make up a large share of biogas plant costs. The investment costs of a biogas plant naturally depend on its size (capacity and throughput). Biogas installations follow economies of scale, meaning that as the capacity of a plant increases, the specific investment costs decrease. Plant capacities are, however, limited by available substrates and infrastructure; this means that the lower specific costs associated with larger-scale plants are sometimes not achievable.
Investment costs: Investment costs (depreciation costs) including peripheral equipment and installations make up a large share of biogas plant costs. The investment costs of a biogas plant naturally depend on its size (capacity and throughput). Biogas installations follow economies of scale, meaning that as the capacity of a plant increases, the specific investment costs decrease. Plant capacities are, however, limited by available substrates and infrastructure; this means that the lower specific costs associated with larger-scale plants are sometimes not achievable.
Moreover, some investment costs are feedstock-dependent. Biogas plants for the treatment of municipal wastes are more expensive because they have large closed halls (e.g., with low pressure to reduce smells). These halls are used for the handling and pre-treatment of feedstocks, with impurities (packaging, plastics, metal, etc.) being separated from the organic matter. Investment costs for energy crop digesters or industrial wastewater treatment reactors are often lower, as they need fewer peripheral installations. Another substantial part of the costs can be the biogas utilisation. From an investment perspective, direct heat utilisation is the cheapest solution. In industrial settings the biogas produced can often be co-fired in gas burners, with no or limited adaptation needed. More sophisticated gas utilisation concepts (e.g., combined heat and power production, upgrading to biomethane, fuel cells) result in higher investment costs.
Staff costs: Today, state-of-the-art biogas plants are well automated, resulting in fairly low staff costs; however, extensive feedstock pre- treatment—as occurs with the organic fraction of municipal solid waste or packaged food waste—can increase the demand for human labour.
In general, biogas technology, and in particular the microbial degradation process, is rather complex; it is thus clearly advantageous to have well-trained staff to guarantee a stable, reliable operation.
Digestate logistics costs: The digestate produced can generate considerable costs, depending on the size of the installation and how integrated it is into local organic fertiliser utilisation. The most common digestate utilisation is land application as fertiliser, but this is limited because there may not be farmland nearby and/or because of environmental legislation (e.g., the EU Nitrate Directive). This means that in very large-scale biogas installations, the digestate land application can become a bottleneck because the transport distances to agricultural land where the digestate can be applied increases with the size of the installation, and so too do the transportation and application costs. In small-scale biogas plants–especially in an agricultural setting–the digestate application as a fertiliser on the land is often not a problem. Large digestate storage facilities are typically needed, as land application can be prohibited during winter, the agricultural soils cannot effectively take up nutrients and digestate must be stored.
Other costs: These are costs for maintenance of the machinery, property, insurance, administration, etc. The following figure shows the typical cost distribution of German biogas plants.
The following figure shows the typical cost distribution of German biogas plants.
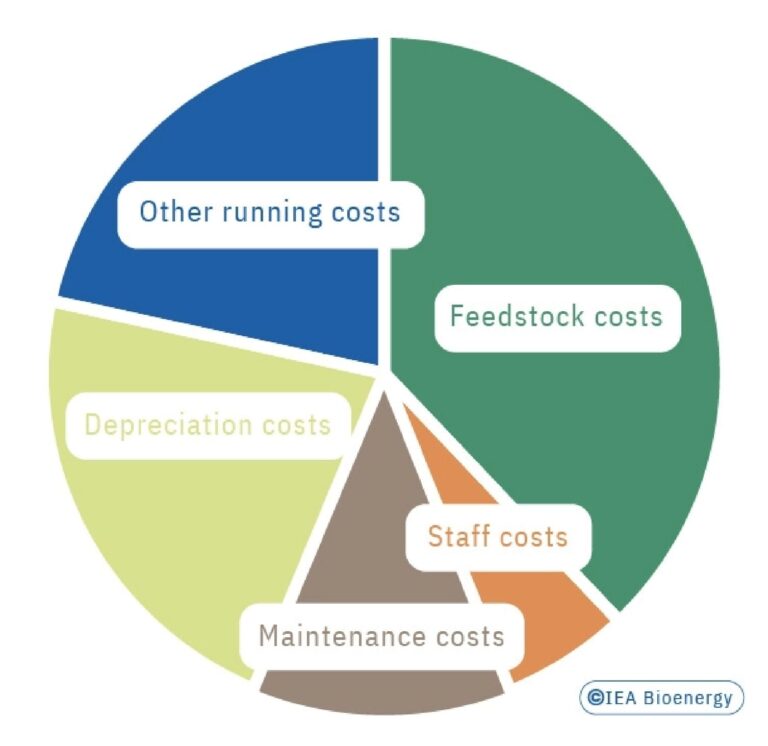
Current research gaps and opportunities
Biogas production provides a wide range of opportunities. It is a mature technology that can be applied for a large range of feedstocks. It can be very effectively linked to agriculture and food processing or wastewater treatment. It not only contributes to energy provision, but also effectively addresses environmental challenges (waste treatment, nutrient management, and emission reduction). It also supports rural development.
A recent trend has been the push towards greening gas grids and consequently increasing biomethane production in biogas plants. Examples from Sweden show that biomethane can also play an important role in the transport sector, for both light- and heavy-duty vehicles.

Biogas technology has the following aspects which still have potential for optimisation:
Systems integration: A promising approach for innovative biogas projects is the system integration of a plant into its surroundings and its specific boundary conditions. As projects differ from case to case, different aspects of biogas technology can be more attractive. An important example is the ability to ramp production up and down in relation to demands from the power grids (where intermittent renewables like solar and wind are playing an increasing role). Biogas technology is also easy to combine with biorefinery concepts for the treatment of intermediates or residues or with power-to-gas concepts in combination with renewable hydrogen.
Feedstock pre-treatment: Different technologies can be applied for the pre-treatment of biogas feedstocks: mechanical, thermal, microbial, enzymatic, and combinations of these. Pre-treatment has two aims: to break down feedstocks/feedstock components that were not previously microbially degradable; and/or to decrease degradation time. As feedstock pre- treatment often increases investment costs and energy input, it has to be verified for every specific case/feedstock that pre-treatment is efficient and advantageous.
Digestate treatment: In large-scale biogas applications the digestates (fermentation residues) can create a bottleneck; as land applications are limited by regulations (e.g., the European Nitrate Directive), large quantities of digestate may need to be transported over long distances for application. A great deal of R&D activity is taking place to investigate and optimise the treatment or processing of digestate. These processes are converting digestate into a nutrient- rich concentrate (that is more economical to transport over large distances) and process water.
Digestion of industrial wastes: During large-scale industrial processes, specific residues or waste waters often occur. The anaerobic digestion process has to be tailored to treat the specific substrates and counteract inhibitions or deficiency in trace elements.
Economically viable small-scale biogas plants: As feedstock availability limits the capacity of biogas plants, small-scale plants tend to have high specific costs due to economy of scale. For a sustainable utilisation of substrates and their integration into agricultural infrastructures, economic solutions for small plant capacities (e.g., 50–200 kWel) are needed.
Biogas plants as biorefineries: The biogas process not only has the potential to produce energy: intermediate products such as carboxylic acids can be valorised directly as chemicals.

Powerful policy instruments
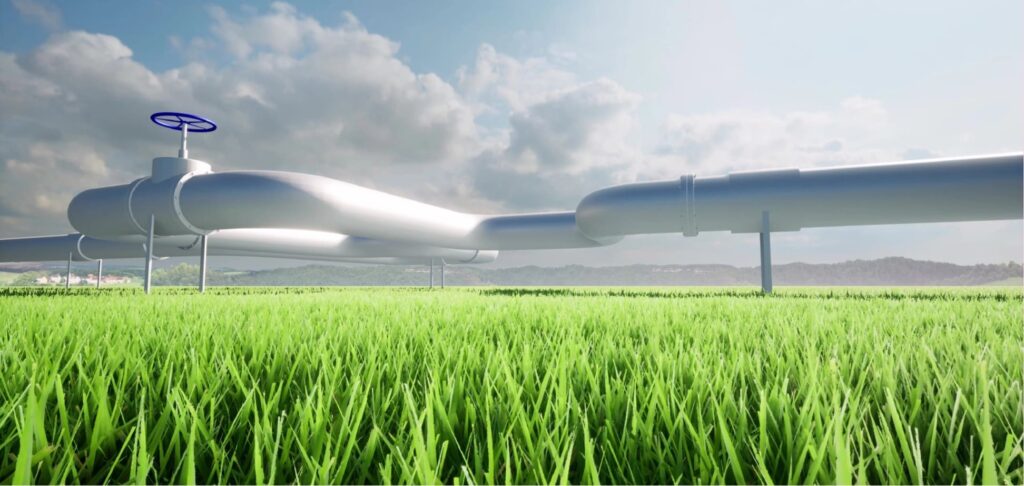
In the vast majority of cases, the implementation of biogas is dependent on government incentives. The reasons for supporting biogas production and use are diverse: production of renewable energy (power, biomethane); reducing negative environmental impacts (e.g., avoiding methane emissions); and achieving a circular economy together with sustainable food production or waste treatment. The general idea is to provide incentives that reflect the actual costs of investment and long-term operation of the renewable gas industry; this can ensure bankability for the developer and a price-effective market environment for the user of renewable gas. Several different approaches can be used to support biogas development:
Clear framework policies: Roadmaps need to be created for renewable gas development, including for substrate availability, development costs, defined time-specific targets as a portion of energy use, and what infrastructure is required and/or already available. Unnecessary barriers and inhibitory regulations need to be eradicated as much as possible at the technical and regulatory level.
Waste regulations: Specific waste regulations can also encourage increased biogas production.
Landfill bans support the biological treatment of organic waste in, for example, biogas plants. As a result, gate fees are provided to the waste treatment facility.
For the direct financial support of biogas production (or utilisation) the following examples can be given:
- Feed-in tariffs: In many countries feed-in tariffs guarantee a higher price for the energy provided by biogas plants.
- Specific investment subsidies: A share of the investment costs is covered by the funding institution.
- Quotas: These place an obligation on fuel providers to ensure that renewable fuels supply a minimum proportion of the fuel market; quotas are a very effective policy tool for avoiding price competition between renewable gas and fossil gas.
- Tax exemptions: Support can also be provided by granting tax exemptions to the biogas sector. In Finland, Norway, and Sweden, for example, these tax exemptions are directed to users of biogas.
- Carbon price: Avoided CO2 (GHG) emissions must have a sufficient monetary value associated with them; a realistic carbon price would stimulate development and drive the transformation of green gas while encouraging competition between renewable technologies in specific sectors, which should lead to the phase-out of specific incentives.
- IEA Bioenergy Task 37 (2009) Biogas upgrading technologies – developments and innovations” LINK accessed 18/02/2022
- IEA Bioenergy Task 37 (2020). Integration of Biogas Systems into the Energy System: Technical aspects of flexible plant operation, LINK accessed 18/02/2022
- Bischofsberger W, Dichtl N et al. Anaerobtechnik, 2nd ed.; Springer: Berlin/Heidelberg, Germany, 2005; pp. 396–417LINK accessed 27/06/2022
- IEA Bioenergy Task 37 (2021) Perspectives on biomethane as a transport fuel within a circular economy, energy, and environmental systemLINK accessed 27/06/2022
- IEA Bioenergy Task 37 (2017) Methane emissions from biogas plants – Methods for measurement, results and effect on greenhouse gas balance of electricity produced LINK accessed 18/02/2022
- IEA Bioenergy (2020) Advanced Biofuels – Potential for Cost Reduction LINK accessed 18/02/2022
- Montgomery L, Bochmann G (2014): Pretreatment of feedstock for enhanced biogas production , 24, IEA BioenergyLINK accessed 18/02/2022
- Drosg B, Fuchs W et al.(2015) Nutrient Recovery by Biogas Digestate Processing, 40, IEA BioenergyLINK accessed 18/02/2022